Organic Reactions:
Organic reactions are chemical reactions involving organic compounds. The basic organic chemistry reaction types are addition reactions, elimination reactions, substitution reactions, pericyclic reactions, rearrangement reactions, photochemical reactions and redox reactions. In organic synthesis, organic reactions are used in the construction of new organic molecules. The production of many man-made chemicals such as drugs, plastics, food additives, fabrics depend on organic reactions.
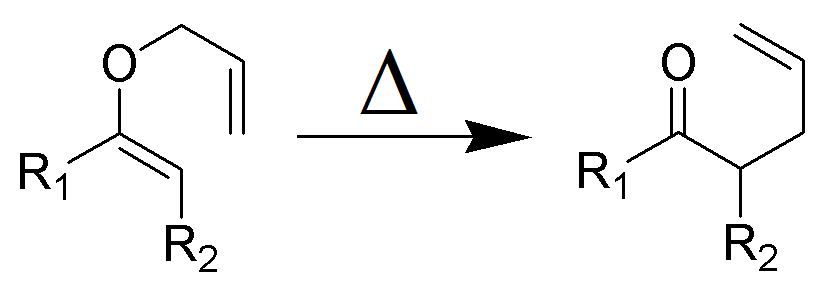
Common Classes of Organic Reactions:
Substitution Reactions:
In a substitution reaction, one atom or a group of atoms in a substance is replaced by another atom or group of atoms from another substance. A typical substitution reaction is reacting the hydroxide ion with methyl chloride:

Methyl chloride has a polar C–Cl bond, with the carbon atom having a partial positive charge. In Equation -3, the electronegative Cl atom is replaced by another electronegative species that is a stronger nucleophile, in this case OH−. Reactions of this sort are called nucleophilic substitution reactions. For this type of reaction to occur, the nucleophilic reactant must possess a pair of electrons and have a greater affinity for the electropositive carbon atom than the original substituent.
One type of nucleophilic substitution reaction is shown in Equation -3. It proceeds by a mechanism in which the lone pair of electrons on the entering nucleophile (OH−) attacks the partially positively charged carbon atom of the polar C–Cl bond, causing the C–Cl bond to weaken and break:
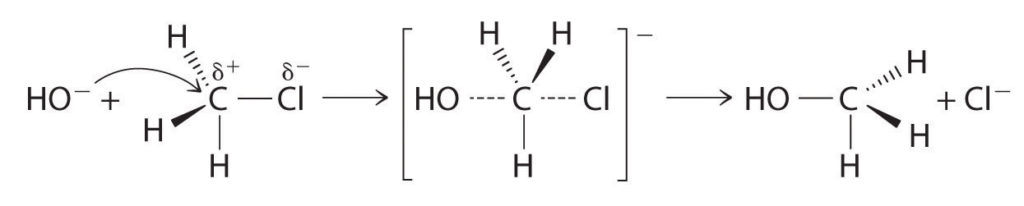
Elimination Reactions:
Some reactions involve the removal, or “elimination,” of adjacent atoms from a molecule. This results in the formation of a multiple bond and the release of a small molecule, so they are called elimination reactions. They have the general form
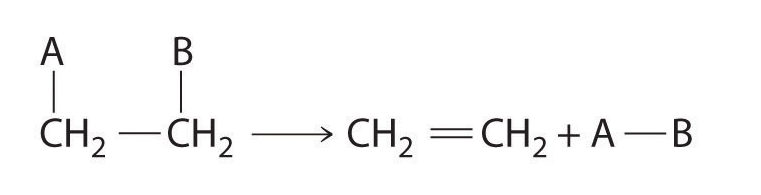
and are similar to cleavage reactions in inorganic compounds. A typical example is the conversion of ethyl chloride to ethylene:

Addition Reactions:
A reaction in which the components of a species A–B are added to adjacent atoms across a carbon–carbon multiple bond is called an addition reaction. An example is the reverse of the reaction shown in Equation -6, reacting HCl with ethylene to give ethyl chloride:

Although a multiple bond is stronger than a single bond, the π bonds of the multiple bond are weaker than the σ bond. The high electron density located between multiply bonded carbon atoms, however, causes alkenes and alkynes to behave like nucleophiles, where nucleophilic attack occurs from the more weakly bound π electrons. Hence alkenes and alkynes are regarded as functional groups. Nucleophilic attack occurs on the Hδ+ atom of the polar HCl bond, initially producing a species with a carbon that has only three bonds, a carbocation. In a second nucleophilic attack, Cl−, the electrophile in Equation-7, attacks the carbocation:
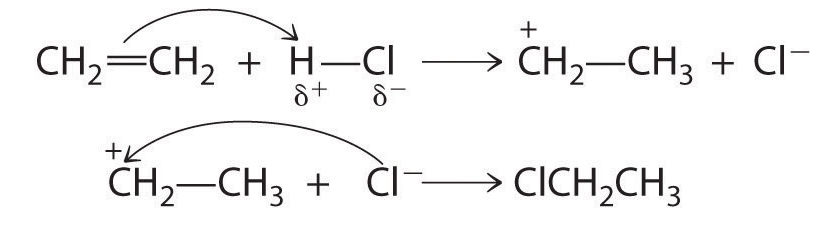
Alcohols, an important class of organic compounds, are often produced by addition reactions. Initial attack by the π bond of an alkene on a Hδ+ of H3O+ produces a carbocation. The carbocation then undergoes nucleophilic attack by a lone pair of electrons from H2O followed by elimination of H+ to form the alcohol.
Radical Reactions:
Many important organic reactions involve radicals, such as the combustion of fuels. Probably the best known is reacting a saturated hydrocarbon, such as ethane, with a halogen, such as Br2. The overall reaction is as follows:
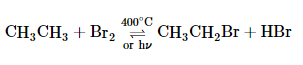
Radical chain reactions occur in three stages: initiation, propagation, and termination. At high temperature or in the presence of light, the relatively weak Br–Br bond is broken in an initiation step that produces an appreciable number of Br atoms (Br·). During propagation, a bromine atom attacks ethane, producing a radical, which then reacts with another bromine molecule to produce ethyl bromide:
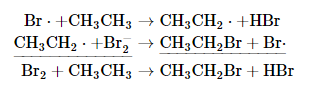
The sum of the two propagation steps corresponds to the balanced chemical equation for the overall reaction. There are three possible termination steps: the combination of (1) two bromine atoms, (2) two ethyl radicals, or (3) an ethyl and a bromine radical:
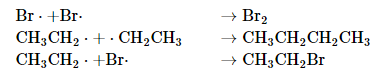
Because radicals are powerful nucleophiles and hence highly reactive, such reactions are not very selective.
For example, the chlorination of n-butane gives a roughly 70:30 mixture of 2-chlorobutane, formed from the more stable radical by reacting a secondary carbon and 1-chlorobutane.
Oxidation–Reduction Reactions:
Oxidation–reduction reactions, which are common in organic chemistry, can often be identified by changes in the number of oxygen atoms at a particular position in the hydrocarbon skeleton or in the number of bonds between carbon and oxygen at that position. An increase in either corresponds to an oxidation, whereas a decrease corresponds to a reduction. Conversely, an increase in the number of hydrogen atoms in a hydrocarbon is often an indication of a reduction. We can illustrate these points by considering how the oxidation state of the carbon atom changes in the series of compounds, which is shown in part (a) in Figure 1(a).
The number of oxygen atoms or the number of bonds to oxygen changes throughout the series. Hence the conversion of methane to formic acid is an oxidation, whereas the conversion of carbon dioxide to methanol is a reduction. Also, the number of hydrogen atoms increases in going from the most oxidized to least oxidized compound. As expected, as the oxidation state of carbon increases, the carbon becomes a more potent electrophile. Thus the carbon of CO2 is a stronger electrophile (i.e., more susceptible to nucleophilic attack) than the carbon of an alkane such as methane.
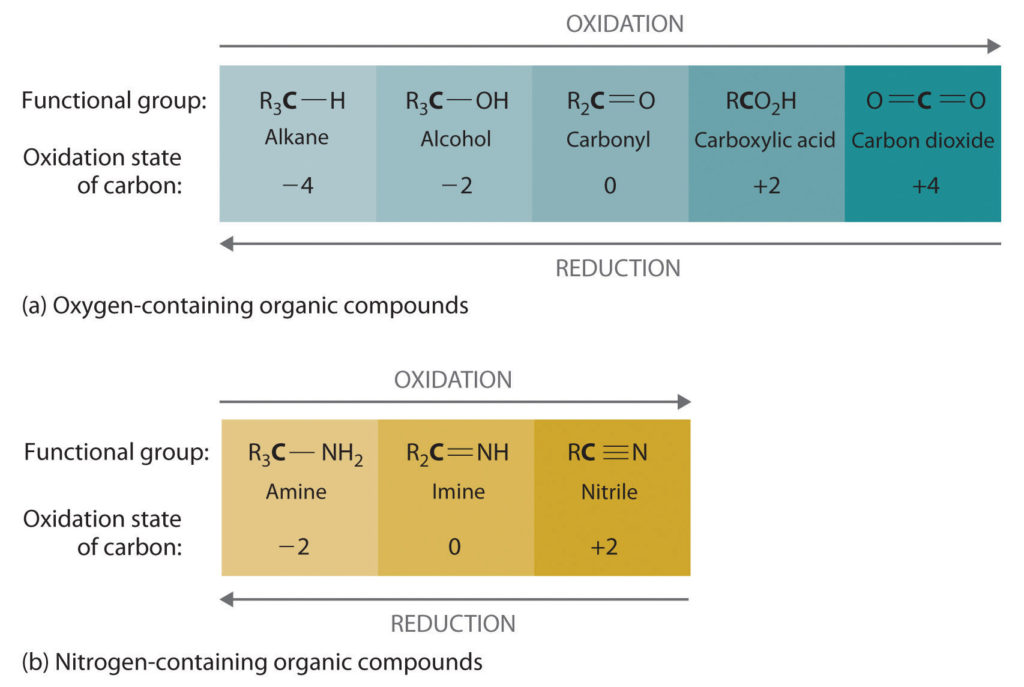
Similarly, in compounds with a carbon–nitrogen bond, the number of bonds between the C and N atoms increases as the oxidation state of the carbon increases (part (b) in Figure 1(a). In a nitrile, which contains the –C≡N group, the carbon has the same oxidation state (+2) as in a carboxylic acid, characterized by the –CO2H group. We therefore expect the carbon of a nitrile to be a rather strong electrophile.